Biochar & Bio-oil
This report was last updated in January 2023. The prior version of this report was published in November 2022.
Summary
Biochar is a charcoal-like substance that is created by heating up biomass (typically agricultural residues) at very high temperatures in a low-oxygen environment – a process known as pyrolysis. Converting biomass into biochar effectively halts much of the decomposition of biomass that would have led to the release of carbon dioxide (CO2) and other greenhouse gases. Biochar is typically applied to soils where it is claimed to enhance soil quality, though this is highly dependent on several conditions that still need to be fully understood through large scale field trials. Since biochar is often sold to farmers as soil amendments, it can be difficult to ascertain the additionality of buying biochar offsets. It is also difficult to quantify the permanence of biochar, as well as its soil benefits, as it can vary based on production and post-production conditions. We believe biochar has the potential to be a beneficial tool in addressing climate change, and have based our recommendations on the projects that we feel best address issues of additionality and permanence. We recommend one biochar project—Mash Makes—which can meet these criteria by giving away biochar we estimate as high-permanence for free.
Bio-oil, a dense liquid that is also created through pyrolysis, can overcome biochar's additionality and permanence challenges if it is not sold for commercial purposes but is instead injected underground in geologic formations. We recomend one company—Charm Industrial—that produces bio-oil and injects it underground.
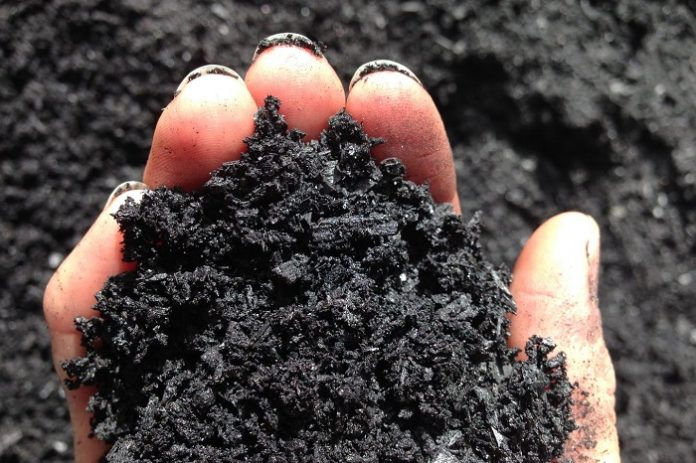
What is biochar?
Carbon removed from the atmosphere through the process of photosynthesis is released back into the atmosphere when biomass (organic material) biologically degrades. Biochar is a charcoal-like product that is created by heating biomass to very high temperatures in a low-oxygen environment—a process known as pyrolysis. Converting biomass to biochar slows the decaying process and locks stored carbon in place, in turn slowing down its release back into the atmosphere.[1] This charcoal-like substance can be added to soils, which prolongs carbon storage, enhances soil quality, and potentially increases crop yields in some conditions, enabling the soil to sequester more carbon overall.[2] A process known as “fast pyrolysis” can be used to convert biomass into bio-oil which can be used as an energy feedstock or pumped back underground for permanent storage.
Biochar as a carbon removal
While biochar is a relatively mature product, its use as a carbon removal is quite nascent. The IPCC revised its carbon removal guidelines to include biochar in 2019.[3] Shortly after, Puro.Earth released its biochar methodology for use in verifying biochar projects in the carbon removal market, followed by the release of the Verra biochar methodology in 2022.[4] Projects are primarily certified by either the European Biochar Certificate or the Puro Standard, with carbon credits available for purchase through traditional carbon registries, marketplaces such as Puro.Earth, and directly from projects themselves.[5]
In fact, at time of writing this overview, major carbon offset registries had not yet begun listing biochar projects. The American Carbon Registry rejected an effort to approve a methodology for biochar projects after a peer review process found limited evidence of the stability of soil carbon sequestration in fields treated with biochar. Another registry, Verra, only recently proposed a methodology for establishing project baselines and project additionality for biochar projects with public review and final approval of this methodology expected by the end of 2021. Despite the lack of existing certification, marketplaces like Puro.Earth have made it possible to buy biochar carbon removal credits from providers outside of traditional carbon registries, and a few biochar and bio-oil producers make it possible to purchase carbon removal credits directly.
Mechanism
When biomass is converted to biochar, it dramatically slows the release of carbon into the atmosphere by preventing the biological decomposition of biomass - avoiding the release of greenhouse gases. For example, projects that collect biologically degrading agricultural wastes and convert them into biochar are generally seen as emissions avoidance projects. Some biochar advocates claim that removing crop waste from natural cycles of growth and decay (by converting it to biochar) is the equivalent of a carbon-negative technology. From our perspective, we admit that is somewhat of a grey area between avoided emissions and CO2 removal. As the production of biochar results in carbon being removed from the atmosphere overall, we lean towards considering biochar and bio-oil projects to be carbon removal, addressing ambiguity by labeling it a medium-permanence removal strategy.
Projects that grow plants for the purposes of converting plant biomass to biochar have a more solid claim to be considered CO2 removal, as the project boundary includes the removal of carbon from the atmosphere through photosynthesis. Growing plants for this purpose, however, can have negative environmental and land use implications, and we therefore believe this approach should only be pursued if it does not harm food security, biodiversity, and rural livelihoods in the process.
Proponents have also claimed that biochar applied to soils can increase crop yields, suggesting higher carbon dioxide uptake by plants through the process of photosynthesis, and therefore that biochar adds to carbon removal from the atmosphere. This effect has not been widely studied in field trials and varies significantly due to soil type, biochar composition, and other environmental conditions (see Causality section). We therefore ignore this in determining whether a biochar project is an avoidance or removal project unless strong evidence can be provided on the added soil carbon sequestration claims.
Causality
Produced from organic materials that have high carbon content, biochar is made up of anywhere between 35% to 95% carbon.[6] The product fixes carbon that would have otherwise been released into the atmosphere as carbon dioxide (every ton of carbon fixed in biochar results in 3.61 tons of avoided CO2 emissions as CO2 has a higher molecular weight than carbon).These organic materials, typically crop residues like wheat straw, corn stover, almond shells, rice husk, and others, would have been broken down by soil microorganisms, which release CO2 and other gases in the process and return nutrients from crop residues back into the soil.[7] A recent study found that leaving crop residues to decay on agriculture farms may actually store more carbon in the soils than would have been the case had the residues been cleared (though these benefits may be offset by increased emissions of nitrous oxide, a strong greenhouse gas).[8] Converting forestry and crop residues to biochar and applying them to soils leads to longer, more stable storage of carbon in soils than if residues were burned or simply applied to soils (approximately 50% of the original carbon is stored in biochar compared to 3% retained after burning and less than 20% after 5-10 years of biological decomposition).[9] The production of bio-oil through fast pyrolysis that is subsequently sequestered into injection wells also results in a more stable storage of carbon than would have otherwise decomposed.
Biochar's and bio-oil's ability to fix carbon that would have otherwise more quickly decomposed is assessed as high, though the longevity of this benefit varies based on several factors, which are covered in the Permanence section.
Project Additionality
Biochar is a product that is commonly sold to farms where it is added to soils. Biochar projects that primarily depend on the sale of carbon credits, as opposed to the sale of physical biochar to farms, can make a reasonable claim to additionality. These projects tend to provide biochar to end users for free or at a substantial discount. Bio-oil that is sequestered underground and not sold to an end user would also satisfy additionality.
Many of the projects we evaluated, however, depend primarily on the sale of biochar to farms as a soil amendment, making it hard to determine whether the biochar would have been produced without the sale of credits. For example, in 2012, it was found that an estimated 90% of biochar produced in Europe has been used as a feed additive in livestock farming for years, suggesting an already robust existing market for the product.[10] A 2018 producer survey estimated total biochar production at 36,700 to 76,600 tons per year in North America. A separate 2018 analysis estimated global biochar production at over 394 kilotons in 2021, which is expected to reach 781 kilotons by 2028.[11] Determining the additionality of credits that support an already growing industry will require market analyses that can isolate growth in biochar production based on demand for credits. The ability to purchase biochar credits is relatively new, thereby limiting robust analysis of their additionality. A techno-economic analysis of biochar production cited wholesale biochar prices as being between $600/ton to $2,778/ton of biochar, with the most commonly cited sales price being $1,600/ton of biochar.[12] This would correspond to a carbon price of $237 to $1100/ton of CO2e, assuming a 70% carbon content. Biochar credits sold for substantially less than this carbon price are likely to be heavily dependent on end user sales, suggesting lower levels of project additionality. However, this is an admittedly crude approach to estimating additionality.
With the exception of projects that do not generate profits from the sale of biochar or bio-oil project additionality for biochar is typically assessed as low.
Marginal Additionality
The production of biochar depends on a number of key inputs, including energy for the pyrolysis process, biomass feedstock, and pyrolysis units to conduct pyrolysis. The degree of marginal additionality depends on how constrained biomass producers are in obtaining these key inputs in response to revenue from credit sales. Biochar producers who are able to easily access new biomass feedstocks like agriculture waste residues, or are able to easily deploy new, modular pyrolysis units can make a strong case for marginal additionality. Alternatively, biochar producers that struggle to source new biomass feedstocks or depend on large, industrial scale pyrolysis units have a lower degree of marginal additionality. Overall, biochar projects tend to have high marginal additionality unless a project is unable to readily acquire new pyrolysis capacity or biomass feedstock because of credit sales.
Permanence
Converting biomass feedstock into biochar fixes carbon that would have otherwise been released into the atmosphere as the original biomass degrades. There are a number of factors that affect the stability, or durability, of the carbon in biochar: (i) biochar characteristics, (ii) production conditions, and (iii) post-production conditions. A combination of these factors determines the overall permanence of a biochar project; we expand upon each of these further in Appendix 1.
(i) Biochar characteristics
Molar ratios: the ratio of H:C(org) and O:C(org) in biochar determines its stability. For medium permanence biochar (with a half-life of 100-1000 years under laboratory conditions), the O:C(org) ratios should be between 0.2-0.6. For high permanence biochar (with a half-life greater than 1000 years under laboratory conditions), the ratio should be less than 0.2.
Fixed carbon content: this is the proportion of stable carbon in biochar. The higher this number is, the more carbon can be stored per tonne of biochar.[13]
(ii) Production conditions
Pyrolysis temperature: Pyrolysis temperature is the temperature at which feedstock is converted to biochar. Pyrolysis temperatures of over 500°C to 600°C are most effective in producing stable biochar; however, this is also influenced by the type of feedstock.[14]
Feedstock: Feedstock (the material being used to create biochar) also affects the permanence of biochar. Woody feedstocks tend to be the most stable, and grassy and manure feedstocks the least.[15] Crop waste and grassy feedstocks are intermediates between the two but can have wide variability in their stability depending on their ash content; low ash crop wastes such as sugar cane and nut shells are more suitable for carbon storage than rice husks and straws.[16]
(iii) Post-production conditions
The real-world applications of biochar can also strongly determine its stability in soils. The different geographic regions in which biochar is applied contain a diversity of soil types, moisture levels, mineral contents, and microorganism conditions, which can have varied impacts on biochar carbon decomposition.[17] More research and field trials are needed to achieve greater precision around biochar’s durability in soils.
Carbon stored in bio-oil that is injected into injection wells is even less likely to decompose than biochar applied to soils. This is primarily because bio-oil sinks to the bottom of injection wells, whereas biochar is distributed near soil surface where it interacts with soil microbes and other elements. Injection wells are regulated by the US Environmental Protection Agency (EPA) and are used to inject hazardous or non-hazardous wastes and fluids in deep geologic formations. While carbon stored in bio-oil that is injected this way is less reversible than biochar in soils, this storage method is not without its risks. Lax oversight into over 680,000 underground waste and injection wells in the United States could lead to leaks and structural failures, however the EPA has deemed the probability of well failures to be low. In addition, bio-oil is denser than other brines, oils, and gases typically stored in these formations, and has been shown to form a solid approximately 48 hours after injection.[18] Alongside EPA monitoring bolstered by recent airborne leakage sensor technology, this gives us higher confidence in the permanence of bio-oil.[19]
While the permanence of bio-oil is generally assessed to be in the medium range (100+ years), the permanence of biochar should be assessed on a case-by-case basis.
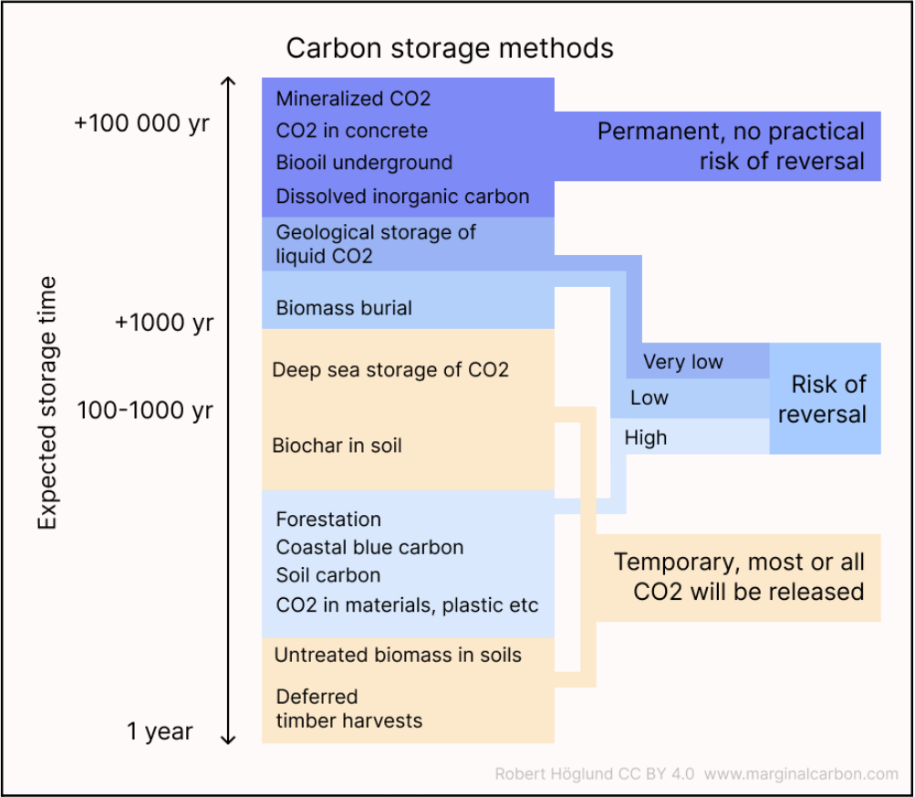
Figure 1. Carbon storage methods by expected storage time, including biochar in soil in the 100-1000 yr range and bio-oil underground in the +100,000 yr range.
Cost
Puro Earth, a marketplace listing 23 biochar projects as of this writing, lists prices for biochar-based carbon removal credits ranging from $98 (100 EUR at the time of writing) to $524 (535 EUR) per ton of CO2. This is substantially more expensive than traditional avoidance-based carbon offset projects, but are cheaper than some of the technological carbon dioxide removal projects like direct air capture. One reason for the cost disparity between projects is that some biochar providers (like CarboCulture and HUSK Ventures) make their biochar available to farmers at a significant discount and depend more heavily on the sale of credits, and are therefore pricing their credits higher. While we believe biochar projects that rely primarily on revenues from credit sales have a stronger case for additionality, they are often high cost. Projects that produce bio-oil from biomass that is sequestered underground (not sold) are priced even higher than biochar credits and are also considered high cost. For projects that sell to farmers and use carbon credits to lower the cost marginally, their “true” cost is difficult to ascertain, since one would need to understand the additional amount of biochar demanded due to the cost difference. We at Giving Green value when organizations are financially transparent so that additionality can be better assessed.
Co-benefits
When added to soil in some conditions, specific types of biochar can create long-term carbon pools in the soil, stimulate microbial benefits, increase soil’s water holding capacity, improve nutrient availability, decrease susceptibility to plant disease, and increase crop yields.[20] However, these benefits depend on a variety of environmental factors and biochar characteristics; we expand further upon these in Appendix II.
Environmental factors
The impact of applying biochar to soil on increasing crop yields depends on a number of environmental factors like soil type, soil pH, fertilizer inputs, and soil fertility.[21] Biochar could cause a positive increase in crop yields where it is able to improve the water holding capacity of the soil or add nutrients.
Biochar characteristics
Feedstocks that are less suited to carbon sequestration appear more beneficial for increasing agricultural outputs, due to their high mineral and unstable carbon content. Lower pyrolysis temperatures (between 401°C and 500°C) also had the greatest positive effects on crop yield.[22]
While these are helpful guidelines, additional long-term field studies on the effects of biochar in soils under different contexts are needed to address our uncertainty regarding the co-benefits of biochar purposed for carbon sequestration. In the US, the recent Biochar Research Network Act of 2022 will establish a national biochar research network to quantify the characteristics that influence biochar co-benefits and carbon sequestration and understand the trade-offs between these uses.
Conclusion
We believe that biochar and bio-oil hold significant promise as valuable tools in addressing climate change, especially given their ability to store carbon for 100+ and 1000+ years, respectively. However, there can be a great deal of variance regarding the additionality, permanence, and co-benefits. In order to ensure that the climate potential of these pathways is maximized, we think a project-by-project assessment is necessary.
At this time we recommend one biochar project, Mash Makes; it is able to address uncertainty around additionality and permanence by providing its biochar—which we estimate as being high permanence—to farmers for free, and it is currently undertaking field trials to assess co-benefits. We also recommend one bio-oil provider, Charm Industrial; it produces bio-oil and sequesters it underground, addressing some of the additionality and permanence issues with biochar providers.
Glossary
Aromatic compound: Flat, cyclical chemical compounds containing aromatic rings, which are a highly stable chemical structure.
Inorganic carbon: Carbon-containing compounds that lack carbon-hydrogen bonds; these are more stable than organic carbon-containing compounds
Feedstock: The biological material used to create biochar.
Labile carbon: The residues of volatile or semi-volatile components of biochar:, the non-stable parts of organic carbon that can be easily degraded by microorganisms over weeks to months. This is not made up of aromatic compounds.
Organic carbon: Carbon-containing compounds that have carbon-hydrogen bonds present; these are less stable than inorganic carbon-containing compounds.
Pyrolysis: Thermal decomposition of materials at elevated temperatures.
Appendix I - Biochar Characteristics
(i) Biochar characteristics
Molar ratios
Hydrogen to organic carbon molar ratios (H:C(org)) and oxygen to organic carbon molar ratios (O:C(org)) are useful for identifying high-permanence biochars. The lower the ratio, the more stable the final product is and the longer it will take to degrade.[23] Where possible, search for H:C(org) ratios over O:C(org) ratios due to their greater accuracy.[24] Puro Earth’s and the European Biochar Certificate (EBC)’s methodology requires H:C(org) ratios of less than 0.7 and O:C(org) molar ratios less than 0.4, which is the point at which the biochar is stable enough to use in carbon storage.[25]
For medium permanence biochar (with a half-life of 100-1000 years under laboratory conditions), the O:Corg ratios should be between 0.2-0.6. For high permanence biochar (with a half-life greater than 1000 years under laboratory conditions), the ratio should be less than 0.2.[26]
Fixed carbon content
Fixed carbon content is the proportion of stable carbon in biochar, which is also important to consider. This is a measure of how much carbon the biochar can store, showing the carbon sequestration potential per tonne of biochar.[27] Puro Earth requires a fixed carbon content of over 50%, but the higher this number is, the more carbon can be stored per tonne of biochar.[28]
(ii) Production conditions
Pyrolysis temperature
Pyrolysis temperature is the temperature at which feedstock is converted to biochar. Higher temperature pyrolysis is strongly correlated to lower molar ratios, higher fixed carbon content, and greater biochar stability.[29] While Puro Earth standards allow for pyrolysis temperatures ranging from 350°C to 1000 °C, higher pyrolysis temperatures indicate better quality, more stable biochar. Pyrolysis temperatures of over 500°C to 600°C are most effective in producing stable biochar; however, this is also influenced by the type of feedstock.[30]
Feedstock
Feedstock (the material being used to create biochar) also affects the permanence of biochar. Different feedstocks have different permanence depending on their structural stability, ash content, and H:C(org) and O:C(org) ratios.[31] Feedstocks can be classified as woody (softwood, hardwood), crop waste (e.g. corn, wheat straw, and rice straw/husk), grassy, and manure (often poultry, pig, and cattle).[32] Woody feedstocks tend to be the most stable, and grassy and manure feedstocks the least.[33] Crop waste and grassy feedstocks are intermediates between the two but can have wide variability in their stability depending on their ash content; low ash crop wastes such as sugar cane and nut shells are more suitable for carbon storage than rice husks and straws.[34]

Figure 2: SD, RH, FW, PL and PS indicate biochar of sawdust, rice husk, food waste, poultry litter and paper sludge and adjacent numeric values 1, 2, 3 and 4 indicate different pyrolysis temperatures of 350 °C, 450 °C, 550 °C, 650 °C respectively.[35]
(iii) Post-production conditions
The real-world applications of biochar can also strongly determine its stability in soils. The different geographic regions in which biochar is applied contain a diversity of soil types, moisture levels, mineral contents, and microorganism conditions.[36] The decomposition rates of stable carbon remain relatively unchanged by different post-production conditions, but the unstable portion is highly affected by these. Soil type influences how quickly unstable carbon in biochar degrades, with clay soils stabilizing soil carbon faster than mineral or sandy soils.[37] The mineral content of the soil also factors in, with soils high in iron and low in calcium promoting biochar stability.[38] Temperature is thought to have only minimal effects on biochar decomposition rates; even under climate warming scenarios, soil temperatures are not expected to become high enough to impact carbon stability in biochar.[39] However, abrupt soil moisture increases (from flooding or heavy rainfall) can rapidly increase the decomposition rate of unstable biochar; low moisture levels should be maintained where possible.[40]
Soil microorganisms also play a significant role in biochar decomposition; conditions that enhance microbial activity can decrease the lifespan of biochar in soils.[41] High amounts of unstable carbon encourage microorganism activity, this can be remedied through high pyrolysis temperature and woodier feedstocks.[42] Biochars that have a high portion of unstable carbon from low pyrolysis temperatures and manure feedstocks can therefore temporarily enhance carbon loss. Ultimately, post-production conditions matter most for biochar with high unstable carbon content, and the net benefit on soil carbon storage is thought to be positive in the long term regardless.[43] Estimates of >1000-year half-life times for high-quality biochar remain accurate in temperate climates, but more research is needed on half-lives in a tropical context. However, any differences between laboratory and field estimates are likely to be greatest amongst lower quality biochars with high proportions of unstable biochar.[44] More research and field trials are needed to achieve greater precision around biochar’s durability in soils.
Appendix II - Biochar Co-benefits
Environmental Factors
The impact of applying biochar to soil on increasing crop yields depends on a number of environmental factors like soil type, soil pH, fertilizer inputs, and soil fertility.[45] A meta-analysis on crop yields found that in tropical climates, where acidic, nutrient-poor, soils are more common, biochar elicited a 25% increase in yield, but had almost no effect in temperate climates.[46] Another meta-analysis found the effects of biochar on crop yields ranging from a reduction in crop yields by 28% to increases of 39%. Their results reinforce that positive effects on crop yields are most common in soils that are either acidic or neutral, or those that have a coarse to medium texture. This suggests that biochar increases crop yields where it is able to improve the water holding capacity of the soil or produce a liming effect (where calcium and magnesium are added to decrease soil acidity and add nutrients).[47] Positive effects may be further reinforced when biochar is applied alongside an inorganic fertilizer, which can increase yields by a further 10%.[48]
Biochar characteristics
There are two main characteristics of biochar that influence its suitability for agricultural use: feedstock and pyrolysis temperature, which determines organic carbon and mineral content, pH, and surface area.[49] Feedstocks that are less suited to carbon sequestration appear more beneficial for increasing agricultural outputs, due to their high mineral and unstable carbon content. In a 2022 meta-analysis, high-nutrient, manure-based biochars resulted in the greatest crop increases; lower-nutrient woody and crop-waste biochars still provided positive effects but performed significantly lower. Lower pyrolysis temperatures (between 401°C and 500°C) also had the greatest positive effects on crop yield, with no significant effect on yield observed when pyrolysis temperatures were greater than 600 °C.[50] This is because the mineral compounds that form at higher temperatures can be inaccessible for plant uptake.[51]
While these are helpful guidelines, additional long-term field studies on the effects of biochar in soils under different contexts are needed to address our uncertainty regarding the co-benefits of biochar purposed for carbon sequestration. The recent Biochar Research Network Act of 2022 will establish a national biochar research network to quantify the characteristics that influence biochar co-benefits and carbon sequestration and understand the trade-offs between these uses.
Endnotes
1. See “What is Biochar?” American University, n.d.
2. “Here we use a global-scale meta-analysis to show that biochar has, on average, no effect on crop yield in temperate latitudes, yet elicits a 25% average increase in yield in the tropics.” Jeffery et al., 2017.
3. See Appendix 4. IPCC, 2019.
4. Puro Standard Biochar Methodology. Puro.earth, 2022.; M0044 Methodology for Biochar Utilization in Soil and Non-Soil Applications, v1.0. Verra, 2022.
5. Puro Standard. Puro.Earth, n.d., European Biochar Certificate. European Biochar Certificate 2022
6. See section 7.1, European Biochar Certificate 2022
7. “The decomposition of crop residues releases nutrients for plant use”. Rakkar et al., 2018.
8. “In fact, the researchers estimate that leaving crops residues to rot on the ground enables carbon to be locked into the soil for four times longer than if these residues were cleared away.” Bryce, 2021; “Assessments of the GHG balance of cropping systems have shown that the N2O emissions associated with crop residue decomposition can, in some situations, offset the positive effects that the recycling of crop residues has on maintaining or increasing soil C stocks”. Lashermes, 2022.
9. See abstract. Gaunt & Rondon, 2006.
10. “90% of the biochar produced in Europe is used in livestock farming.” Gerlach & Schmidt, 2012.
11. See ‘Producers Survey Results’. Groot, 2018; “In terms of volume, the market was sized at 394.09 kilotons in 2021 and is expected to reach 781.09 kilotons by 2028.” Triton Market Research, 2022.
12. See Table 4. Biochar Prices Reported in Literature. Nematian, Keske & Ng'ombe, 2021; “Reported prices paid for biochar ranged widely depending on the packaging and volume
13. See section 1.1.1., Puro Earth 2021
14. See ‘Carbon, hydrogen, and oxygen’ section, Ippolito et al, 2020
15. See abstract and conclusions, Hassan et al. 2020
16. See section 3.1.2., Conz et al. 2017; section 3.1., Leng & Huang, 2018; ‘The amounts and rates of biochar decomposition’, Wang et al., 2015
17. See ‘Future perspective’, Spokas, 2010; ‘Abiotic factors and processes’, Wang et al. 2015.
18. “After injection, the bio-oil sinks within the formation. Even better, the bio-oil has tendency to polymerize into a solid, and at the temperatures and pressures in these formations, our surface-level 3rd party lab experiments have shown that bio-oil becomes a solid, locked in place, in just 48 hours”. Charm, n.d.
19. “Inexpensive and precise aerial rapid screening of methane leaks could be a proverbial game-changer for environmental monitoring.” Myers, 2022.
20. See section 2.6.1. CDR Primer, n.d.
21. See section 3.2.2. Adhikari et al., 2018.
22. See sections 4.1 and 4.3. Bai et al., 2022.
23. H:Corg and O:Corg molar ratios that use organic carbon content should be used instead of H:C and O:C molar ratios that measure total carbon content where possible, as they are a more reliable indicator of permanence. This is because inorganic carbon does not form part of the stable aromatic compound structure that constitutes biochar, and thus, will not be as permanent. This is of particular importance for high-ash biochars (such as poultry manure or paper mill waste) that contain a higher proportion of inorganic carbon. See section 3.1.1, 3.1.2., Leng et al. 2019;
24. H:Corg is considered a better measure than O:Corg as it can be measured directly, whereas O:Corg has to be derived from other measurements (O = 100 − C − H − N − S − ash) and so is often overestimated, see introduction in Klasson 2017 and section 3.1.2., Leng et al. 2019:
25. See 1.1.6., Puro.earth 2022; section 7.2 &7.3, European Biochar Certificate 2022; Appendix 7, International Biochar Initiative 2015; introduction, Klasson 2017
26. See conclusion, Spokas 2010.
27. See section 4.1.1., Leng et al. 2019.
28. See section 1.1.1., Puro Earth 2021
29. See section 3.2., McBeath et al. 2015; section 3.3., Enders et al. 2012.
30. See ‘Carbon, hydrogen, and oxygen’ section, Ippolito et al, 2020
31. See abstract, introduction, materials and methods Chaturvedi et al. 2020; ‘Biomass chemical characterization’, Veiga et al. 2017; section 3.1., Enders et al. 2012;
32. See ‘Feedstock choice’ section, Ippolito et al, 2020
33. See abstract and conclusions, Hassan et al. 2020
34. See section 3.1.2., Conz et al. 2017; section 3.1., Leng & Huang, 2018; ‘The amounts and rates of biochar decomposition’, Wang et al., 2015
36. See ‘Future perspective’, Spokas, 2010; ‘Abiotic factors and processes’, Wang et al. 2015.
37. See highlights, Fang et al. 2015; conclusions and implications, Fang et al. 2013.
38. See conclusion, Yang et al. 2021.
39. See “Temperature sensitivity of biochar decomposition” section. Lehmann et al. 2012.
40. See conclusion, Yang et al. 2021.
41. See ‘Biological decomposition’ Lehmann et al. 2012; ‘Microorganisms and biochar stability’, Ameloot et al. 2013
42. The portion of labile (unstable) carbon in biochar is determined largely by pyrolysis temperature and feedstock type. Higher pyrolysis temperatures and feedstocks with higher lignin content (hard woods) will lead to a lower portion of labile carbon. See ‘Influence of native SOM: priming and co-metabolism’, Ameloot et al. 2013; section 4.1, Zimmerman et al. 2010.
43. Biochars that have a high portion of labile carbon can enhance carbon mineralisation in soil (the process of turning the carbon in organic matter into carbon dioxide) in early stages, facilitating carbon loss. See ‘Influence of biochar production and application conditions” Ameloot et al. 2013; introduction & conclusion Zimmerman et al. 2010.
45. See section 3.2.2. Adhikari et al., 2018.
46. “ Here we use a global-scale meta-analysis to show that biochar has, on average, no effect on crop yield in temperate latitudes, yet elicits a 25% average increase in yield in the tropics.” Jeffery et al., 2017.
47. “The greatest (positive) effects with regard to soil analyses were seen in acidic (14%) and neutral pH soils (13%), and in soils with a coarse (10%) or medium texture (13%). This suggests that two of the main mechanisms for yield increase may be a liming effect and an improved water holding capacity of the soil, along with improved crop nutrient availability.” Jeffery el al., 2011.
48. “Biochar + inorganic fertilizer increased yield by an additional 10%.” Bai et al., 2022.
49. See ‘Biochar: Production and Characteristics’. Al-Wabel et al., 2017.
50. See sections 4.1 and 4.3. Bai et al., 2022.
51. “Specifically, with increasing pyrolysis temperature one typically observes increasing biochar C, P, K, Ca, ash content, pH, specific surface area (SSA), and decreasing N, H, and O content.” Ippolito et al., 2020.